Planetary Insights – “Polluted” White Dwarfs
Planetary Profiles – Michael Jura
Rheasilvia basin generated from Dawn topographic data.
By Jennifer Scully
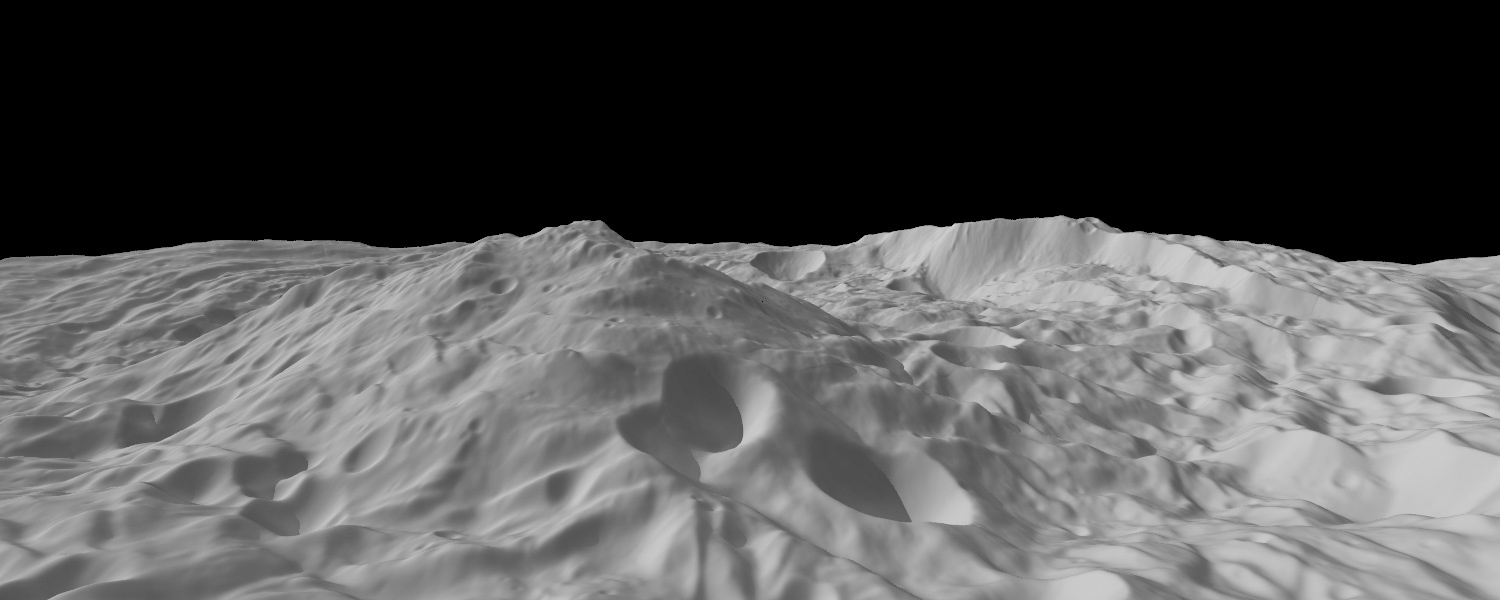
The above image was generated from Dawn topographic data and is a tilted view of Vesta’s Rheasilvia impact basin, which is a ~500km depression that dominates the topography of Vesta’s southern hemisphere.
The Dawn spacecraft arrived at the asteroid Vesta on 16th July 2011, with the aim to understand the evolution of Vesta. Since then it has been acquiring data with its three different instruments: the Framing Camera (FC), the Visible and Infrared Spectrometer (VIR) and the Gamma Ray and Neutron Detector (GRaND).
Even before Dawn reached Vesta the Rheasilvia basin was visible in Hubble images of the asteroid (for more information see Thomas, P.C. et al. 1997. Science, 277, 1492-1495). The Rheasilvia basin contains a large central complex, which is visible as the roughly circular mound offset from the center in the anaglyph image below. To create this anaglyph, two differently colored images were superimposed with an offset to create depth. When viewed through red-blue glasses it shows a 3D view of Vesta’s surface.
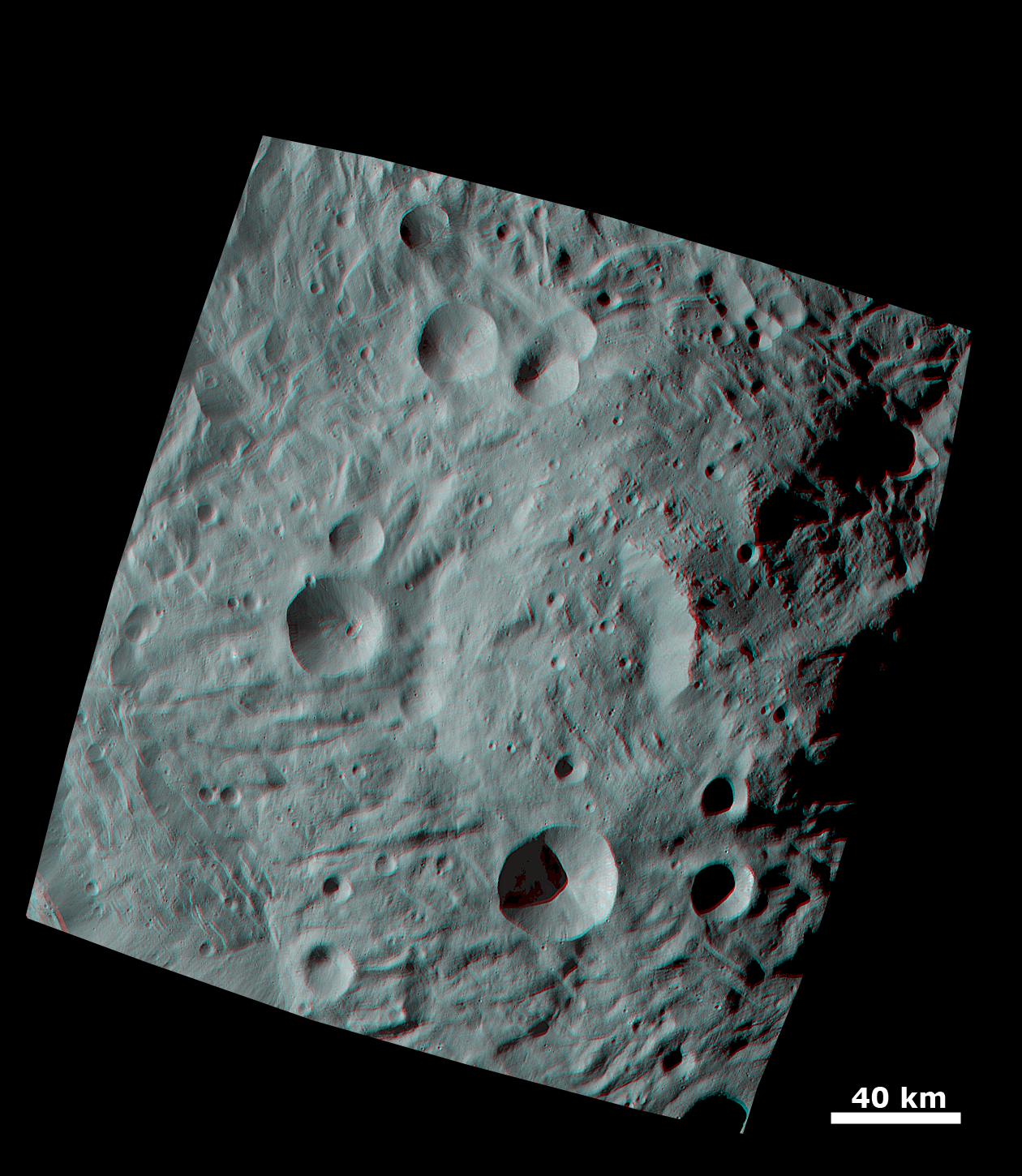
The Rheasilvia central complex is approximately 200km in diameter and has approximately 20km of relief from its base, which makes it about 2.5 taller than Mt. Everest! The topographic differences in the Rheasilvia basin are clear in the cross section in the figure below, which shows the central complex in the foreground and the rim in the background. Also evident is that the rim of the Rheasilvia basin is very pronounced in places. A part of the rim forms a steep cliff, called a scarp, visible in the right side of the figure. There is also a large scarp on the central complex and surrounding the central complex is the hummocky (i.e. wavy/undulating) texture of the Rheasilvia basin. Possible formation mechanisms for this hummocky texture are currently under investigation.
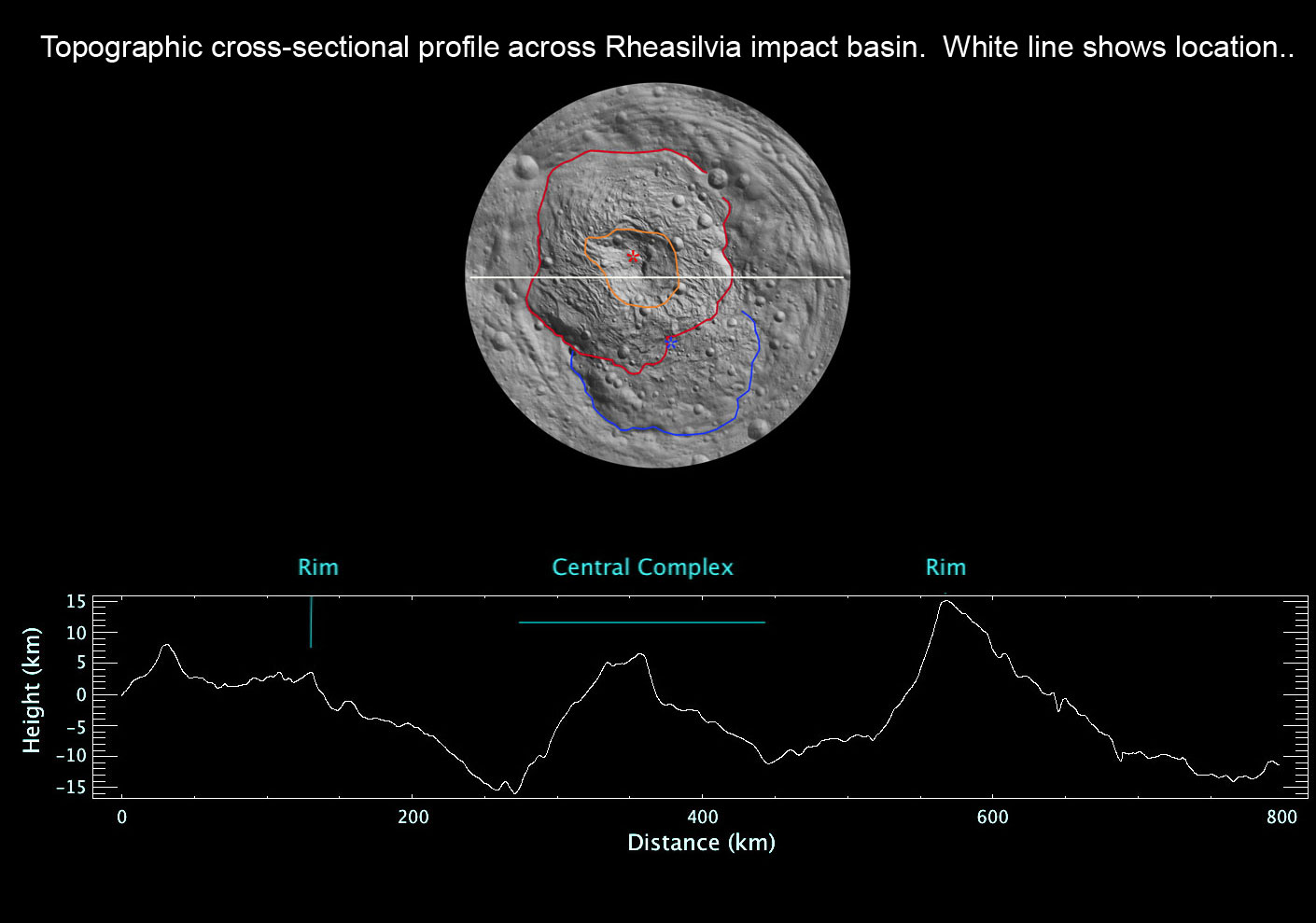
The Rheasilvia basin, and the impact that formed it, is possibly the source of the Vestoid meteorites. The Vestoids are a suit of meteorites believed to come from Vesta because their reflectance spectra match the average reflectance spectrum of Vesta (for more information see McSween, H.Y. et al. 2010. Space Science Reviews, 163, 141-174). For this reason the Dawn mission is unusual because samples of the asteroid were already on Earth before the Dawn spacecraft, or any spacecraft, reached and orbited Vesta. These meteorite samples, combined with the remotely sensed data from Dawn, will help to develop a picture of the origin and evolution of Vesta. It is thought that Vesta formed within the first few million years of the solar system and, unlike the Earth, Vesta has not been subsequently modified by geological and atmospheric processes. Thus, developing a picture of the origin and evolution of Vesta provides a window into the development of the early solar system.
When Dawn leaves Vesta in late 2012 it will travel to the asteroid Ceres. Ceres is the largest asteroid in the asteroid belt and, unlike Vesta, likely contains water ice. Consequently, Vesta and Ceres, which are the most massive asteroids in the solar system, provide insights into the evolution of both the rocky bodies and the icier bodies of the solar system. For more information about the Dawn mission see the paper “The Dawn Mission to Vesta and Ceres” available at http://www.springerlink.com/content/f46t37l0617571j6/. For more images from the Dawn mission see the Dawn Image of the Day website at http://dawn.jpl.nasa.gov/multimedia/imageoftheday.
The Dawn mission to Vesta and Ceres is managed by NASA’s Jet Propulsion Laboratory, a division of the California Institute of Technology in Pasadena, for NASA’s Science Mission Directorate, Washington D.C. UCLA is responsible for overall Dawn mission science. The Dawn framing cameras have been developed and built under the leadership of the Max Planck Institute for Solar System Research, Katlenburg-Lindau, Germany, with significant contributions by DLR German Aerospace Center, Institute of Planetary Research, Berlin, and in coordination with the Institute of Computer and Communication Network Engineering, Braunschweig. The Framing Camera project is funded by the Max Planck Society, DLR, and NASA/JPL.
Cabeus crater, as viewed by the Diviner Lunar Radiometer.
By Michaela Shopland
The Diviner Lunar Radiometer team based at UCLA, played an integral role in the LCROSS experiment – not only did observations from their instrument help in the selection of the impact site, but the data they gathered after impact helped to eventually confirm the presence of water ice in the lunar surface.
LCROSS slammed into the Moon on October 9 2009, in a controlled impact designed to reveal once and for all whether water ice is present beneath its surface. The impact site was situated within a permanently-shadowed part of Cabeus Crater with an average annual temperature of 37 K (-393 °F), making it one of the coldest locations near the lunar south pole. Temperature data from Diviner had played a key role in the selection of Cabeus as the target for LCROSS, and in the lead up to impact, scientists and engineers worked together to ensure that Diviner was able to target the impact site for 8 orbits spaced roughly 2 hours apart, the closest of which passed by 90 seconds after impact.
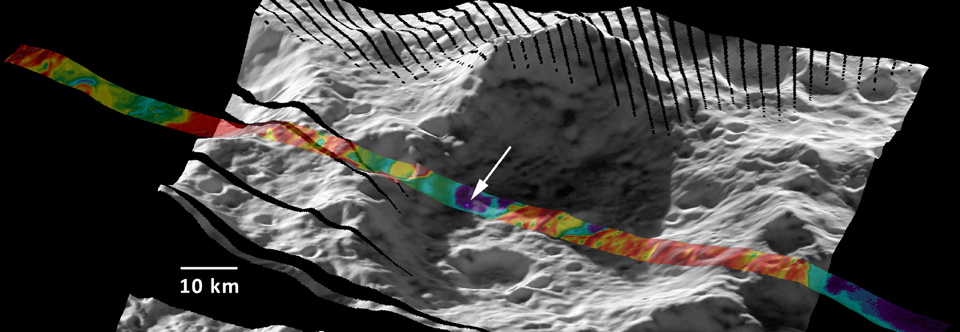
Recent UCLA graduate Paul Hayne was monitoring the data in real-time as it was sent back from Diviner after the impact.
“During the fly-by 90 seconds after impact, all seven of Diviner’s infrared channels measured an enhanced thermal signal from the crater. The more sensitive of its two solar channels also measured the thermal signal, along with reflected sunlight from the impact plume. Two hours later, the three longest wavelength channels picked up the signal, and after four hours only one channel detected anything above the background temperature.”
Scientists were able to learn two things from these measurements: firstly, they were able to constrain the mass of material that was ejected outwards into space from the impact crater; secondly, they were able to infer the initial temperature and make estimates about the effects of ice in the soil on the observed cooling behavior.
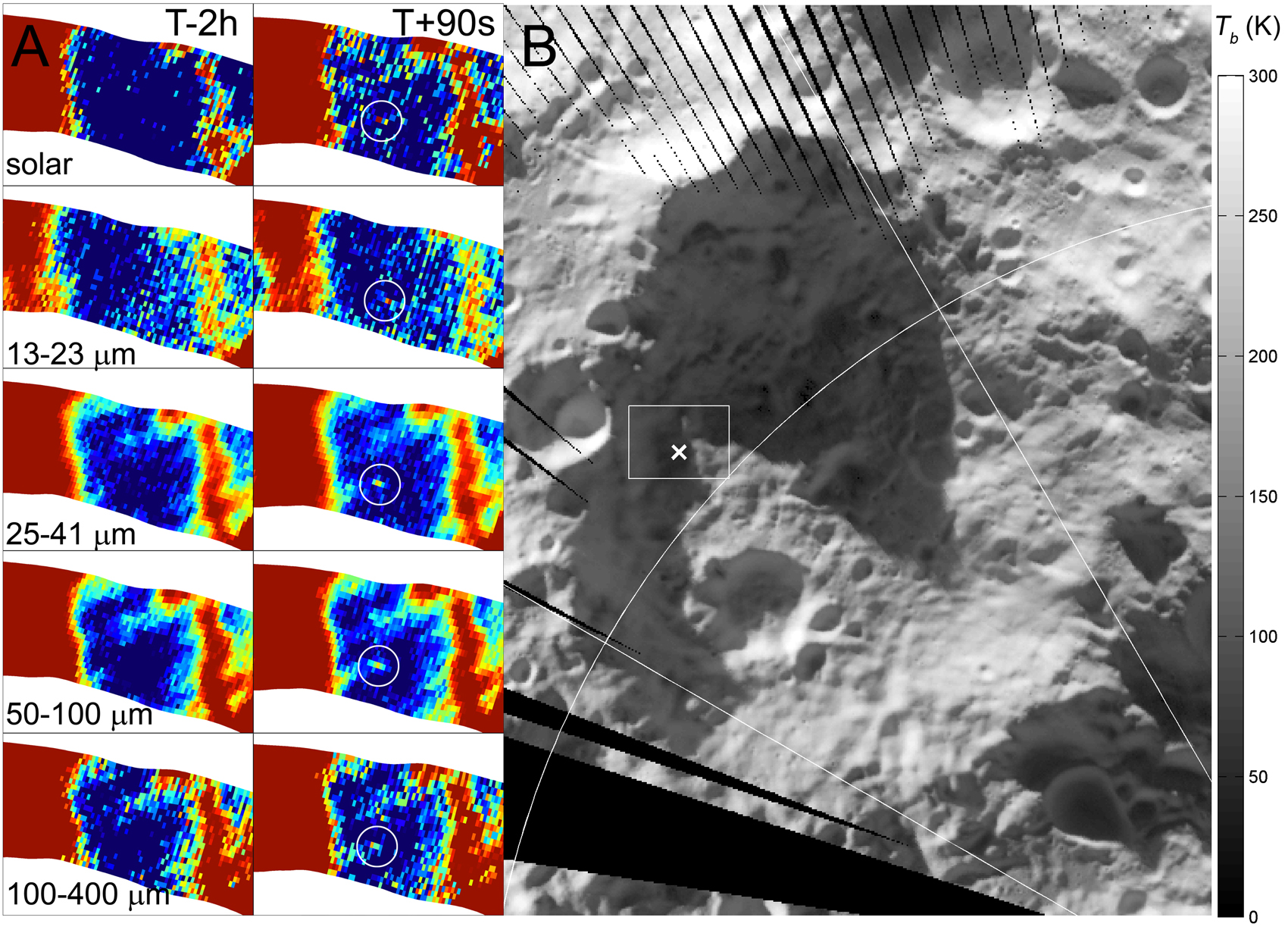
Given that ice within soil pore spaces influences cooling because it uses up heat energy in the process of sublimating, and conducts heat more efficiently than lunar soil does, scientists were able to use Diviner’s measurements of cooling at the impact site to place constraints on the proportion of volatiles present.
“The fact that heated material was still visible to Diviner after four hours indicates LCROSS did not hit a skating rink – the ice must have been mixed within the soil. We estimate that for an area of 30 to 200 m2, the steaming crater could produce more than enough water vapor to account for what was observed by LCROSS over a four minute period.”
The observations provide strong evidence in support of the theory that there are volatiles ‘cold-trapped’ within permanently-shadowed regions on the Moon. The current thinking is that they were delivered there by impacts from icy bodies which themselves originated in the outer solar system.
The Diviner Lunar Radiometer is one of seven instruments aboard NASA’s Lunar Reconnaissance Orbiter. Principal investigator, David Paige, is a professor in the Department of Earth & Space Sciences at UCLA.
A full text version of the article published in Science can be found here:
Disrupted Asteroid P/2010 A2
By David Jewitt
Caption: N = principal nucleus, a 120-meter diameter body. AA and BB = cross arms of the “X” structure, though to consist of larger blocks released from the nucleus. C = objects embedded in the “X” arms and detected on more than one date. These are secondary sources of dust for the tail. E = faint extension above the main tail, slightly blended with a smeared, background galaxy. F = filaments of dust released from discrete sources within the “X”. S = Star and background galaxy trails, imperfectly removed from the data.
P/2010 A2 has the orbit of an asteroid but a physical appearance more like a comet. It is one of the “active Asteroids” (or “main-belt comets”) discovered in the last few years. This object was observed at high resolution using the Hubble Space Telescope from January to May 2010, showing subtle changes associated with changes in the viewing geometry. The object shows a point-like nucleus at the leading end that is about 120 meters in diameter. Most likely, this object was struck by a much smaller asteroid, probably just a few meters across, in early 2009. The dispersing remnant, seen in the picture above, includes ribbons and sheets of debris that are pushed away from the sun by the pressure of sunlight. From models of this radiation pressure, we find that the dust particles in the tail range from centimeter size near the nucleus, N, to millimeter size at the far right edge of the picture.
The scientific significance of this object is that it opens the door to real-time study of asteroid disruptions. In the past, we could observe the ancient remnants of past collisions, but had to resort to elaborate models in the context of modern-day collisional evolution of the belt. Collisions are a primary mechanism of asteroid destruction and an important source of dust.
A paper describing the discovery and HST observations is linked here (1 Mb pdf). Pre-discovery observations are linked here (2.8Mb pdf). There is also a stand-alone P/2010 A2 web site.
Soon after P/2010 A2, a second example of likely impact ejection from an asteroid, this time the 100 times larger body (596) Scheila, was discovered by Steve Larson.
THEMIS measures how solar wind interacts with Earth’s magnetic field
By Christine Gabrielse
Some planets have magnetic fields that act as invisible force fields against the solar wind and the sun’s radiation, called magnetospheres. Although their natural shape is more like a doughnut with the planet at the center, the solar wind is such a strong force that magnetospheres are pulled backwards like a windsock into what we call a magnetotail. The energetic particles carried by the solar wind can sneak into the magnetotail and fill it with energy until an explosive phenomena (“magnetic reconnection”) flings those energetic particles towards the planet. The result is beautiful and dangerous: as the energy hits the planet and creates aurora across the sky, it may also strike orbiting satellites and render them useless. If communication satellites went down at Earth, we could be without GPS and cell phones! I am studying the phenomena that happen when these energetic particles are flung towards a planet in an effort to understand just how they are transported through the magnetotail.
(credit NASA)
http://www.youtube.com/watch?v=l_4tEkwSZhg
(credit SVS/GSFC, J. Raeder/UNH, V. Angelopoulos/UCLA)
Titan, Dione and the ring shepherds Pandora and Pan.
Phobos, moon of Mars, with large crater Stickney on the right
50,000 yr old Meteor Impact Crater near Flagstaff, Arizona.
Meteor Crater (aka Barringer Crater) is about 1 km in diameter and located an hour’s drive east from Flagstaff, Arizona. Formed 50,000 yrs ago by the impact of an iron-nickel asteroid perhaps 50 meters in diameter. Although seen by Barringer as an iron mining prospect, numerous attempts failed to find a buried iron mass from the impactor. We now know that this is because the impact was at a speed high enough to vaporize the projectile as well as a large mass of the target sandstones, causing the crater-forming explosion. Only small fragments of the projectile have been located.
Meteor Crater is especially important in planetary science as it is the prototype structure on which the essential features of craters, generally, were worked out by Gene Shoemaker. Diagnostics of impact include highly shocked minerals (which can only form at pressures created in cosmic impacts) in the crater floor, shatter cones, and an over-turned rim-flap (caused by folding of the pre-impact strata by the blast, a bit like the petals of a giant flower unfolding as they open).
Although small, the impact was no doubt locally devastating to the surrounding plants and animals, showering the environs with hypervelocity rock and metal shrapnel and killing everything for 10’s of kilometers around. The crater is about 200 times smaller than the one responsible for the KT extinction 65 Myr ago. The projectile was similar in size to the one that caused an airburst above Siberia in 1906, but being of iron (instead of stone or ice) was able to deposit its energy into the ground).